Ultimate Issue: Analyzing a Fatal Final Turn
Van’s RV-4 accident presents a tragic case study of the stall-spin scenario.
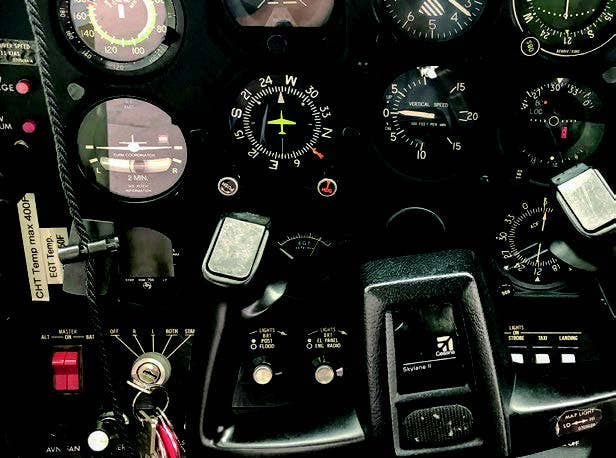
There’s a reason students are taught to establish 1.3 Vs on the downwind leg, begin the descent abeam of the threshold, and maintain a good speed margin throughout the approach. [iStock]
In 1949, the Civil Aeronautics Authority (the precursor to the FAA), reacting to the number of training accidents involving spins, removed the spin from the private pilot syllabus. Some pilots who knew how to spin an airplane suspected that anyone who didn’t wasn’t really a pilot.
Cooler heads observed that the majority of unintentional spins occurred in the traffic pattern, particularly on the base-to-final turn, where there was no room to recover even if the pilot knew how to. So knowing how to spin and recover served no purpose, besides its entertainment value—which, to be sure, was considerable.
Under the new dispensation, pilots were taught, in theory at least, not how to recover from a spin but how to avoid one. Nevertheless, stall spins, usually in the traffic pattern, still account for more than a tenth of all airplane accidents and around a fifth of all fatalities. Because they involve a vertical descent, stall spins are about twice as likely to be fatal as other kinds of airplane accidents.
- READ MORE: A Cautionary Tale About Pilot Freelancing
Why has the FAA’s emphasis on stall avoidance not done more to reduce the number of stall spin accidents? There are probably many reasons, but I think the lack of realism in the training environment deserves some blame. The training stall is a controlled maneuver, briefed in advance, approached gradually, calmly narrated, and recovered from without delay. The real-life, inadvertent stall is sudden, unexpected, and disorienting.
The pilot does not see it coming and so does nothing to prevent it. The training stall is so reassuring that pilots fail to develop a healthy fear of the real thing. After this preamble, you may guess that I am going to talk about a fatal stall spin.
The airplane was a Van’s RV-4, an amateur-built two-seat taildragger with a 150 hp Lycoming engine. It had first been licensed 13 years earlier and later sold by its builder to the 48-year-old pilot, a 1,300-hour ATP with single- and multiengine fixed-wing, helicopter, and instrument ratings. For the past six months, the pilot had been on furlough from regional carrier Envoy Air, where he had logged 954 hours in 70-seat Embraer ERJ-175 regional jets.
On the day of the accident, he added 24 gallons of fuel to the RV and flew from Telluride (KTEX) to Durango (KDRO), Colorado, a 25-minute trip, to pick up a friend. They then flew back to Telluride, where the temperature was 1 degree Fahrenheit, and a 10-knot breeze was blowing straight down Runway 27. The density altitude at the runway was about 9,600 feet.
Entering a wide left-downwind leg at about 100 knots, the pilot gradually decelerated and descended. By the time he began his base-to-final turn, he was about 200 feet above the runway and was going to slightly overshoot the extended centerline if he didn’t tighten his turn. His airspeed dropped to 50 knots, and the airplane stalled and spun. An airport surveillance camera caught the moment—a blur, then a swiftly corkscrewing descent. It was over in a few seconds. Both pilot and passenger died in the crash.
- READ MORE: Two Fatal Cases of the Simply Inexperienced
The National Transportation Safety Board’s finding of probable cause was forthright, though it put the cart before the horse: “The pilot’s failure to maintain adequate airspeed...which resulted in the airplane exceeding its critical angle of attack…” Actually, the opposite happened: The pilot allowed the angle of attack to get too large, and that resulted in a loss of airspeed. It was the angle of attack, not the airspeed, that caused the stall.
Still, it was an airspeed indicator the pilot had in front of him and not an angle-of-attack indicator, so to the extent that the pilot was consciously avoiding a stall, he would have had to use airspeed to do so.
The published stalling speed of the RV-4 at gross weight is 47 knots. In a 30-degree bank, without loss of altitude, that goes up to 50.5. Individual airplanes may differ.
But in any case it’s misleading to make a direct, mathematical link between bank angle and stalling speed, although the NTSB frequently does just that. When you perform a wingover, your bank angle may be 90 degrees, but your stalling speed is certainly not infinite. In the pattern, you can relieve the excess G-force loading associated with banking by allowing the airplane’s downward velocity to increase—assuming that you have sufficient altitude.
On the other hand, with your attention focused on the simultaneous equations of height, position, glide angle, and speed that your mental computer is solving in the traffic pattern, you may not even be aware of a momentary excursion to 1.2 or 1.3 Gs.
The RV-4, with a rectangular wing of comparatively low aspect ratio and no washout, stalls without warning in coordinated flight but is well-behaved and recovers readily. Uncoordinated, it can depart with startling abruptness. It resembles all other airplanes in being less stable when the center of gravity is farther aft, so maneuvering at a speed just a few knots above the stall may be more perilous when there is a passenger in the back seat. Like most small homebuilts, the RV-4 is sensitive to fingertip pressure on the stick and easily overcontrolled.
The NTSB’s report on this accident does not include any information about how many hours the pilot had flown the airplane or how many of those were with a passenger. The FAA registry puts the cancellation of the previous owner/builder’s registration just one month prior to the accident, suggesting the pilot may not have had the airplane for long.
The pilot never stabilized his approach. He descended more or less continuously after entering the downwind leg several hundred feet below pattern altitude—to be sure, the pattern at Telluride is 400 feet higher than normal—and never maintained a steady speed even momentarily. His speed decreased more rapidly as he entered the final turn, perhaps because he felt he was a little too low and instinctively raised the nose. Besides, the terrain rises steeply toward the approach end of Runway 27, possibly making him feel he was descending more rapidly than he really was.
A final factor that may have played a part in this accident is the altitude. The runway elevation at Telluride is at about 9,100 feet. Density altitude doesn’t matter for speed control in the pattern if you pay attention to the airspeed indicator, because all the relevant speeds are indicated airspeeds. But your true airspeed, which is 10 knots greater than indicated, can still create the illusion that you have more speed in reserve than you really do when you are making a low turn to final.
There’s a reason that students are taught to establish 1.3 Vs on the downwind leg, begin the descent abeam of the threshold, and maintain a good speed margin throughout the approach. It helps keep the stall-spin numbers down.
Note: This article is based on the National Transportation Safety Board’s report of the accident and is intended to bring the issues raised to our readers’ attention. It is not intended to judge or reach any definitive conclusions about the ability or capacity of any person, living or dead, or any aircraft or accessory.
This column first appeared in the Summer 2024 Ultimate Issue print edition.

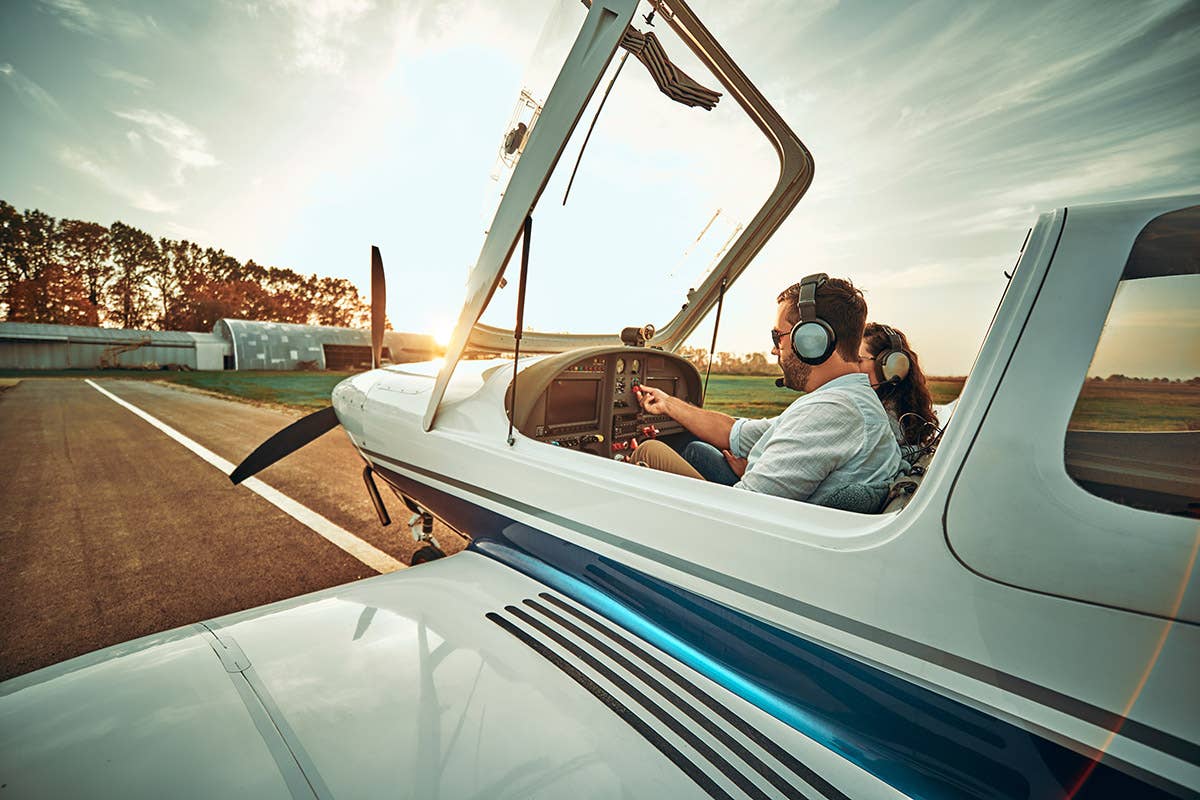
Subscribe to Our Newsletter
Get the latest FLYING stories delivered directly to your inbox